By Seung Ho Lee, Ming Liu, Wasim Saman and Michel Bostrom
Part 2: Economic benefits and field testing results
Abstract
This paper presents a techno-economic evaluation of a phase change material (PCM) thermal battery (TB) system developed through an industry-university cooperation project. The innovative PCM-TB incorporates a newly formulated salt-hydrate PCM with 15˚C phase change temperature (PCT) and an optimised heat exchanger design.
Experimental testing under various real-world conditions was conducted to assess the system performance. In Part 1, the electricity consumption data with and without the TB were generated using simulation software and compared to evaluate its effectiveness. The study also examined the system impact on demand shifting and electricity cost savings using Australia’s time-of-use electricity pricing system.
In this part, Part 2, a cost/benefit analysis of the TB is carried out. Field testing data and economic benefits are evaluated. The field test data further support the positive effects of the TB on building cooling systems. Results indicate that incorporating the TB into the cooling system successfully reduces peak energy demand by 16% and lowers electricity costs by 11%.
TBs show promise for high cooling load applications with significant energy consumption. Further research is necessary to optimise the design parameters and investigate long-term performance and maintenance costs.
Introduction
The first part in this paper introduced the engineering and economic motivation for the use of thermal batteries (TB) in air conditioning systems. It also presented the design details of a prototype TB and its integration into the building air conditioning system. It also described the computer modelling of the whole system integrated into an Adelaide commercial building cooling load data and weather data. The results demonstrated the positive impact of the TB on smoothing the peak electrical demand. In part 2, the economic benefits of using the TB will be presented along with the results of field testing of the TB prototype.
Cost of the PCM-TB and economic benefit
The TB was fabricated using high-quality components and expertise in the field of thermal energy storage. The manufacturer ensured that the TB met all relevant standards and regulations for safety and performance. The total cost of the TB, including a general margin of 15%, was AUD$15,373. This cost includes the heat exchanger, manifold, PCM tank, insulation, and PCM as shown in Table 1. The heat exchanger, manifold, and PCM tank are the most expensive components, accounting for the majority (AUD$6,739) of the total cost. Insulation (AUD$500) is also a crucial component of the system, as it helps to maintain the cold energy stored in the PCM tank. The PCM (AUD$3,373) is another significant cost associated with the system.
The PCM materials used in the system were sourced from China and then mixed locally. The total cost of the TB includes not only the materials and components but also the costs of transportation and installation. Finally, there are other costs associated with the fabrication, transportation, and installation of the TB, including labour, equipment rental, and other miscellaneous expenses. These costs, along with the general margin, account for the remaining AUD$4,731. Overall, the TB provides an effective and cost-efficient solution for the existing cooling system.
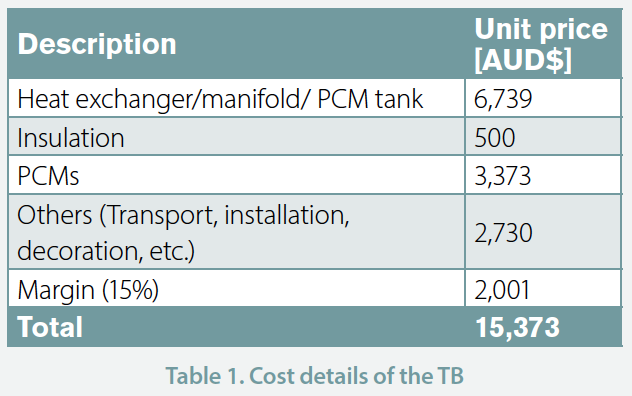
The integration of the TB into the building cooling system resulted in an estimated AUD$1,132 reduction (Figure 8 (a)) in electricity costs by applying the ToU electricity pricing structure, which includes rates of 14.43 cents/kWh during off-peak and 40.52 cents/kWh during peak. Assuming no change in electricity tariff in subsequent years, the simple payback period (PBP) for the TB is approximately 13.6 years.
However, considering the significant rise in electricity prices in Australia over the past decade, as illustrated in Figure 5, and factoring in a 9.0% annual increase in electricity price along with an average interest rate of 1.74% in the subsequent years, the dynamic payback period (PBP) for the TB is approximately 9.26 years. Assuming an average lifespan of 30 years, the IRR for the TB would be approximately 6.13% at a constant electricity price. However, when considering a 9% annual increase in electricity price, the IRR jumps to 15.7%.
Another economic benefit associated with integrating the TB is the “avoided cost” that refers to the expenses that are avoided or saved as a result of integration of the TB instead of increasing the cooling capacity of the existing cooling system to cope with peak demand. The total cost of the TB was AUD$15,373, as shown in Table 1.
Industry estimates suggest a cost of approximately AUD$500 per kW of cooling capacity. Considering the initial 15-minute cooling performance (45kW) of the TB, the cost for additional cooling capacity would be approximately AUD$22,500. Thus, the avoided cost of opting for the TB instead of installing an additional cooling system amounts to AUD$7,127 as shown in Figure 8 (b).
It is worth noting that utility companies commonly assess power usage by calculating the average demand over a 15-minute interval, identifying the highest average 15-minute demand within a month as the peak demand. When the avoided costs are taken into account, selecting the TB over an additional cooling system can result in direct payback, and the IRR for the TB would be approximately 69.0%. This indicates that investing in the TB is financially viable and can provide significant long-term savings compared to using an additional cooling system.
Figure 8. Annual electricity cost savings and avoided cost.
In addition, the TB can help reduce the impact of the recent increase in peak loads in the building sector on the maintenance cost of cooling systems. According to a report by the US Department of Energy, installing a correctly sized cooling system can result in a significant reduction of 10–30% in annual energy expenses [19].
However, if a water chiller operates at a high load for an extended period, it can experience wear and tear on its components, resulting in maintenance issues, repairs, and hidden costs. To address this, the TB, coupled with the water chiller, allows the chiller to operate at a reduced load, which can help extend its lifespan and reduce the need for maintenance and repairs.
Maintenance for cooling system represents over 65% of annual facility management costs and can comprise up to 75% of total maintenance costs over the life of the system [6]. Therefore, by reducing the hidden costs associated with maintenance, the integration of the TB may provide a cost-effective long-term solution for cooling systems. The TB can serve as a viable substitute to counterbalance the elevated cooling load as a consequence of increasing peak demand, thereby reducing maintenance costs.
Field test results
The TB has been installed at the PSC since November 22, 2022 as shown in Figure 9. The purpose of the trial is to confirm the effect of the system on reducing deviations in the feed water temperature used to cool the data centre. This is a crucial factor, as temperature deviations can significantly impact the performance and longevity of the computing equipment.
By stabilising the feed water temperature within the desired range, the TB helps to maintain a consistent and optimal operating environment for the data centre, which is essential for high-performance computing and data storage applications. The installation of the TB represents an important step in improving the energy efficiency and sustainability of the PSC, as it allows for more precise control of the cooling process and reduces the overall energy consumption required to maintain an optimal operating environment. The detailed evaluation of the monitoring data will enable researchers to study the impact of the TB on the data centre’s performance and energy consumption, which may lead to further optimisations in the future.
Figure 9. The TB at the Pawsey Supercomputing Centre.
The experiment spanned a duration of 41 days, starting from January 25, 2023, and ending on March 7, 2023. Throughout this period, a total of 41 charging and discharging cycles of the TB were conducted. To charge the TB, water at a temperature of 8.5~9˚C was used for average 3.5 hours, while discharging took approximately 3 hours.
In the absence of the TB, the 16˚C-loop delivered water to the data centre following the indicated flow path of ③→④→⑦. The charging process followed the path of ①→②. Given that the TB constitutes only a small portion of the total cooling capacity of the existing system, the primary water flow is directed towards the 16˚C-loop (③→④), and only a small fraction of the heated water from the data centre is routed to the TB (⑤→⑥). Subsequently, the cooled water from both the 16˚C-loop and TB merged and were supplied to the data centre (⑦). During the experiment the water temperature at ⑦ was measured in 15 minutes intervals.
For this field test, the CV method (Equation (3) was used to compare the deviation of two feed water temperatures into the PSC, one with TB working and one without. The CV is a statistical measure of the relative variability of a dataset, calculated as the ratio of the standard deviation (STD) to the mean. This method is useful for comparing the deviation of two feeding water temperatures with different means and sample sizes.
The feed water temperature without the TB had an average of 15.94°C and a standard deviation of 0.19, resulting in a CV of 1.19. The feed water temperature with the TB had an average of 15.99˚C and a standard deviation of 0.16, resulting in a CV of 1.02, as shown in Figure 10.
The lower CV value for the feed water temperature with the TB indicates that the system had a more consistent effect on reducing deviations in the temperature of the water used to cool the data centre compared to when it was not working. This is because the relative variability of the feed water temperature was lower when the TB was operational, with a smaller standard deviation relative to the mean.
Overall, the use of CV methods allowed us to quantitatively compare the variability of the two datasets and determine the effect of the system on reducing deviations in the temperature of the water used to cool the data centre.
Conclusions
This study evaluates the techno-economic feasibility of the TB using 15˚C PCM. The economic evaluation was conducted using a computational method employing a building simulation model with data obtained from an in-situ test building. The building model confirmed that the TB component accurately predicts the performance of the TB based on experimental results. The research results obtained through the building model demonstrate the effectiveness and benefits of integrating the TB into an existing building cooling system.
Significant improvements in the cooling system efficiency, particularly during peak periods, were observed, resulting in a higher coefficient of performance (COP) and reduced energy consumption. By maintaining a smaller temperature difference across the cooling system, the TB will enhance the overall efficiency of the cooling system.
The integration of the TB led to substantial electrical and peak demand savings, showcasing its ability to shift cooling energy consumption from peak to off-peak periods.
From an economic perspective, the cost analysis revealed that integrating the TB provided cost savings in electricity expenses. The dynamic payback period, accounting for variation of electricity prices, indicated a favourable return on investment, particularly when considering the projected annual increase in electricity costs. Furthermore, compared to the cost of installing an additional cooling system to increase cooling capacity for peak demand, the TB proved to be a more cost-effective solution, resulting in direct payback and a high internal rate of return.
Moreover, the field test conducted at the PSC confirmed that the TB helped stabilise the feed water temperature in the data centre, ensuring a consistent and optimal operating environment. By reducing deviations in water temperature, the system contributed to improved performance and longevity of the computing equipment, thereby reducing the need for maintenance and repair costs associated with high-load operation of the cooling system.
Overall, this research demonstrates that the TB offers a promising solution for enhancing the efficiency and sustainability of building cooling systems, particularly in applications with high cooling demand during peak periods. Its ability to shift energy consumption, increase COP, provide cost savings, provide means for better utilisation of variable renewable energy supply and reduce maintenance costs makes it a valuable technology for improving energy efficiency and reducing environmental impact in various sectors. Further research and implementation of the TB in different contexts could lead to broader adoption and greater energy savings in the future.
References
1. Faraj, K., et al., A review on phase change materials for thermal energy storage in buildings: Heating and hybrid applications. Journal of Energy Storage, 2021. 33: p. 101913.
2. Heier, J., C. Bales, and V. Martin, Combining thermal energy storage with buildings – a review. Renewable and Sustainable Energy Reviews, 2015. 42: p. 1305-1325.
3. Vega, M., et al., Life cycle assessment of the inclusion of phase change materials in lightweight buildings. Journal of Energy Storage, 2022. 56: p. 105903.
4. Nijmeh, S., et al., A Technical and Economic Study of a Photovoltaic–phase Change Material (PV-PCM) System in Jordan. Jordan Journal of Mechanical and Industrial Engineering, 2020. 14: p. 371-379.
5. de Gracia, A., et al., Life Cycle Assessment of the inclusion of phase change materials (PCM) in experimental buildings. Energy and Buildings, 2010. 42(9): p. 1517-1523.
6. Yang, L., et al., A comprehensive review on sub-zero temperature cold thermal energy storage materials, technologies, and applications: State of the art and recent developments. Applied Energy, 2021. 288: p. 116555.
7. Erdemir, D., N. Altuntop, and Y.A. Çengel, Experimental investigation on the effect of ice storage system on electricity consumption cost for a hypermarket. Energy and Buildings, 2021. 251: p. 111368.
8. Tan, P., et al., Thermal energy storage using phase change materials: Techno-economic evaluation of a cold storage installation in an office building. Applied Energy, 2020. 276: p. 115433.
9. Rahgozar, S., et al., Economic feasibility of ice storage systems for office building applications: A climate sensitivity analysis. Journal of Energy Storage, 2022. 45: p. 103712.
10. Aschberger, E., PCM Operational Report. 2003, Melbourne City Council
11. Alam, M., et al., Energy saving performance assessment and lessons learned from the operation of an active phase change materials system in a multi-storey building in Melbourne. Applied Energy, 2019. 238: p. 1582-1595.
12. Al-Mudhafar, A.H.N., A.F. Nowakowski, and F.C.G.A. Nicolleau, Enhancing the thermal performance of PCM in a shell and tube latent heat energy storage system by utilizing innovative fins. Energy Reports, 2021. 7: p. 120-126.
13. Yu, S., et al., Bio-based PCM/carbon nanomaterials composites with enhanced thermal conductivity. Solar Energy Materials and Solar Cells, 2014. 120, Part B: p. 549-554.
14. Liu, M., et al., Design of sensible and latent heat thermal energy storage systems for concentrated solar power plants: Thermal performance analysis. Renewable energy, 2019: p. 1-12.
15. Tay, N.H.S., et al., Chapter 7 – Static Concept at University of South Australia, in High Temperature Thermal Storage Systems Using Phase Change Materials, L.F. Cabeza and N.H.S. Tay, Editors. 2018, Academic Press. p. 157-191.
16. O’Neill, A., Australia: Inflation rate from 1987 to 2028. 2023: Australia.
17. Australia, R.B.o., The Australian Economy and Financial Markets; Chart Pack. 2023: Australia. p. 20.
18. AER, Chapter 2 – National Electricity Market, in State of the energy market 2022, A.E. Regulator, Editor. 2022: Australia.
19. Energy, D.o. Heating, Ventilation, and Air Conditioning (HVAC). Residential Program Guide 2023 [cited 2023 11th May]; Available from: https://rpsc.energy.gov/tech-solutions/hvac.
Acknowledgments
The authors would like to express their sincere gratitude to all Isothermix colleagues for their unwavering support and invaluable contributions throughout this research project. Their guidance, encouragement, and assistance were truly invaluable, and their diverse perspectives and insights are duly acknowledged.
The authors are also grateful to all of the industry partners who collaborated with Isothermix and the University of South Australia throughout this project, including System Solution Engineering and the Westside Group. Their willingness to share their expertise, resources, and ideas was invaluable to the success of this research.
About the authors
Dr Seung Ho Lee received his PhD degree in mechanical engineering from the University of South Australia in 2020. His doctoral program involved developing phase change materials for building applications. He is currently working as Chief Scientific Officer at Isothermix and is an ARC Industry Fellow at the University of South Australia. He has more than 15 years’ experience in energy and environmental technology. His specific research interests are developing low-cost, easy-to-apply, and environmentally friendly phase change materials, and modelling thermal batteries and building energy performance using heat storage systems.
Dr Ming Liu received her PhD in mechanical engineering in 2010. She is a Senior Research Engineer at Isothermix and Research Fellow at the University of South Australia. Her specific research expertise and interests lie in developing a cost-effective and high-performance phase change thermal energy storage system, which has the strong potential to reduce energy costs and greenhouse gas emissions in Australia and worldwide. Dr Liu has been conducting research into thermal energy storage since 2005. Her research areas includes material selection and characterisation, prototype testing and thermal performance modelling of thermal batteries and storage systems for a range of applications.
Professor Wasim Saman, F.AIRAH, is Emeritus Professor of sustainable energy engineering and former director of the Barbara Hardy Institute/University of South Australia. His recent roles include Research Leader in the CRC for Low Carbon Living; Leader, UniSA Research Node for Low Carbon Living; and Co-Leader in the Australian Solar Thermal Research Institute. His career has focused on sustainable energy education and industry-targeted research since the 1980s. Professor Saman has played a key role in establishing and leading thermal storage research in using phase change materials as low-cost thermal batteries for a range of applications.
Michel Bostrom is the chair, co-founder and CEO of Isothermix and Ametalin, an Australian manufacturer of building membranes and insulation products. Bostrom has an Honours Degree in Economics from the University of Toronto, and has been involved with building and urban economics for most of his career. He has worked in the financial industry as an analyst and a consultant, as well as working in the public sector, notably as a senior policy analyst in the NSW State Treasury. With a foundation in natural sciences, mathematics and statistics, bostrom is deeply involved with developing new building systems and products.